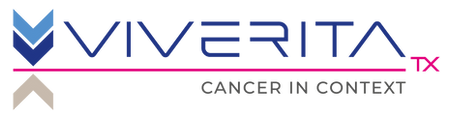
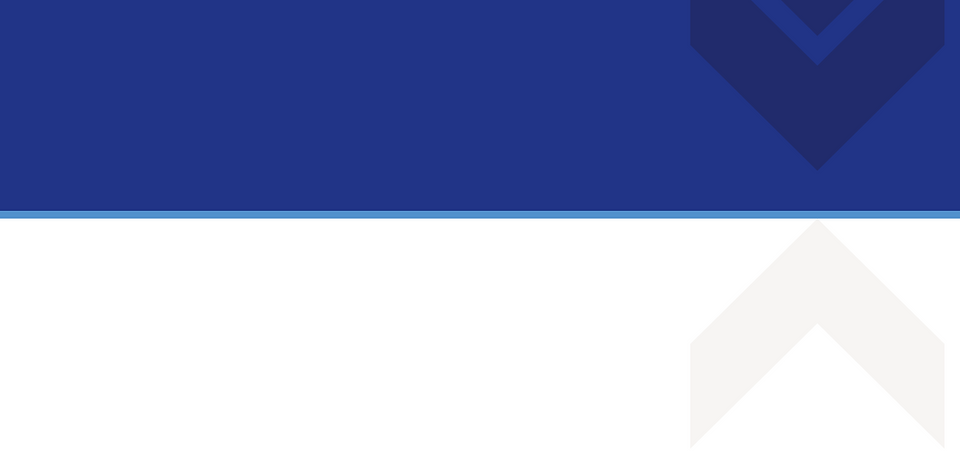
Our Science
Our discovery engine uniquely combines transformative in vivo screening technologies with faithful disease models. This platform allows us to unveil novel target spaces that are inaccessible to others.

CRISPR-StAR (Stochastic Activation by Recombination) transforms in vivo genetic screening. A key limitation with in vivo screening is enormous experimental noise arising from extremely low library coverage and heterogeneous clonal expansion. With internal controls, our CRISPR-StAR technology overcomes this limitation to deliver excellent data quality and, while doing so, with great cost-saving. Read more about this and other transformative CRISPR screening technologies here .
CRISPR screening in Genetically Engineered Mouse Models (GEMM)
Another key limitation with in vivo genetic screening is the lack of faithful disease models. Typically only a few nonrepresentative and aggressively growing mouse syngeneic transplant cancer models are used. These models are already highly transformed and have very limited value in modeling cancer driver gene effects. At ViVerita, we use GEMMs that faithfully model cancer driver gene effects in physiological conditions, allowing us to identify therapeutic targets that specifically block the effects of each cancer driver alteration. Read more about GEMM CRISPR screening in faithful disease models here . We also cross-validate targets identified using GEMMs with patient-derived models.

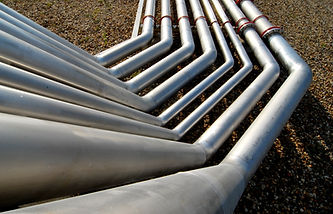
Our Pipeline
We aspire to create novel precision therapies to benefit many more cancer patients. We are building a robust pipeline around high impact novel targets by leveraging our industry-leading in vivo discovery engine and high value targets by using novel therapeutic strategies.
References
Screening technologies:
Uijttewaal ECH, Lee J, Sell AC, Botay N, …, Schramek D, Elling U. CRISPR-StAR enables high-resolution genetic screening in complex in vivo models Nat Biotechnol. 2024 Dec 16. doi: 10.1038/s41587-024-02512-9. https://www.nature.com/articles/s41587-024-02512-9
Michlits, G., Jude, J., Hinterndorfer, M., ..., Elling, U. Multilayered VBC score predicts sgRNAs that efficiently generate loss-of-function alleles. Nat Methods 17, 708–716 (2020). https://www.nature.com/articles/s41592-020-0850-8
Chylinski, K., Hubmann, M., Hanna, R.E., ..., Elling, U. CRISPR-Switch regulates sgRNA activity by Cre recombination for sequential editing of two loci. Nat Commun 10, 5454 (2019). https://www.nature.com/articles/s41467-019-13403-y
Michlits, G., Hubmann, M., Wu, SH., ..., Elling, U. CRISPR-UMI: single-cell lineage tracing of pooled CRISPR–Cas9 screens. Nat Methods 14, 1191–1197 (2017). https://www.nature.com/articles/nmeth.4466
Cancer models:
Martinez, S., Wu, S., Geuenich, M., ..., Schramek, D. In vivo CRISPR screens reveal SCAF1 and USP15 as drivers of pancreatic cancer. Nat Commun15, 5266 (2024). https://www.nature.com/articles/s41467-024-49450-3
Dervovic, d., Malik, AA, ..., Schramek, D. In vivo CRISPR screens reveal Serpinb9 and Adam2 as regulators of immune therapy response in lung cancer, Nat Commun 14, 3150 (2023). https://www.nature.com/articles/s41467-023-38841-7
Loganathan, S., Schleicher, K., ..., Schramek, S. Rare driver mutations in head and neck squamous cell carcinomas converge on NOTCH signaling. Science Vol 367, Issue 6483 (2020), https://www.science.org/doi/10.1126/science.aax0902
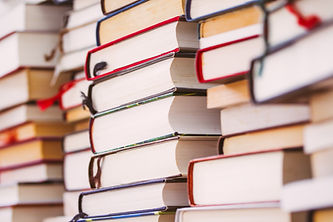